Seaweed Macroalgae Biopolymers: A Sustainable Alternative to Conventional Plastics
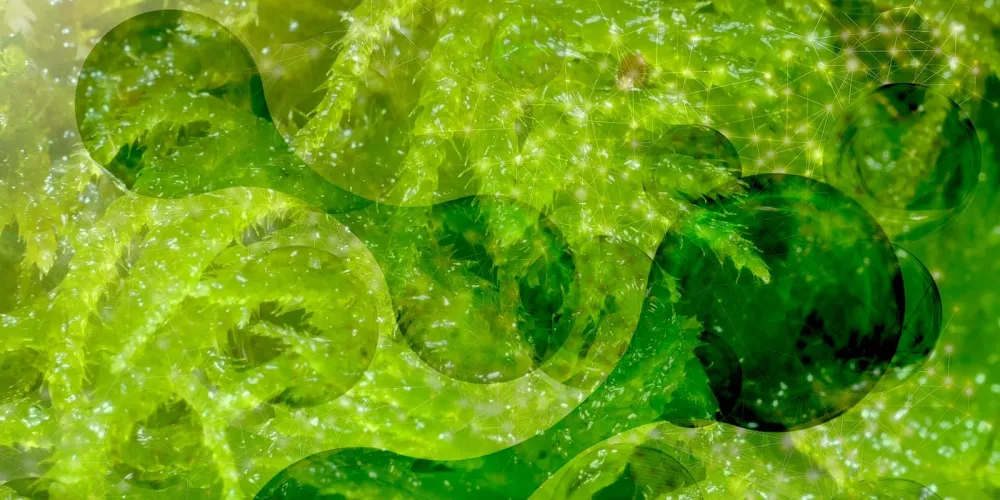
The escalating environmental crisis attributed to plastic pollution has necessitated the exploration of sustainable alternatives. Seaweed macroalgae biopolymers emerge as a promising solution, offering a biodegradable and eco-friendly substitute for conventional plastics. This scholarly article delves into the processes involved in ocean-farming, harvesting, extraction, and processing of seaweed macroalgae biopolymers. It further explores their potential applications in manufacturing sustainable replacements for plastic thin film products, plastic seating utensils, fast food packaging, wearable textiles, and building materials.
The proliferation of plastic waste, particularly in marine environments, has prompted a global search for sustainable materials. Seaweed macroalgae, owing to their rapid growth and minimal requirements for cultivation, present a viable alternative. This article examines the lifecycle of seaweed macroalgae biopolymers from ocean farming to their application in various industries.
Cultivation Techniques
Longline Cultivation
Longline cultivation is a prominent method used in seaweed farming. In this technique, ropes are suspended at an optimal depth in the water column. Seaweed spores are seeded onto these lines, where they grow until ready for harvest. This method is particularly effective in open ocean environments where it leverages the natural flow of nutrients and sunlight. Notable examples include the kelp farms in the coastal waters of Norway, where longline systems have been successfully implemented on a commercial scale.
Off-Bottom Cultivation
Off-bottom cultivation is employed in shallower, coastal waters. In this method, seaweed is grown on nets or meshes that are raised above the seafloor. This technique prevents seaweed from being smothered by sediment and allows for better light penetration and nutrient absorption. A notable case is the cultivation of Ulva (sea lettuce) in the coastal bays of France, utilizing this method for high-yield and quality production.
Integrated Multi-Trophic Aquaculture (IMTA)
IMTA is an advanced method that integrates the cultivation of seaweed with other marine species like fish and shellfish. This symbiotic system allows seaweed to absorb the nutrients released by other organisms, enhancing growth and reducing waste. This method has been successfully demonstrated in the coastal waters of China, where seaweed is grown alongside fish farms, creating a sustainable and eco-efficient system.
Harvesting Methods
Mechanical Harvesting
Mechanical harvesting involves the use of specialized equipment to efficiently collect mature seaweed. This method is particularly effective for large-scale operations. For example, in the Philippines, mechanical harvesters are used for the large-scale collection of Kappaphycus alvarezii, a type of red algae. These machines are designed to cut the seaweed while minimizing damage to the plant and the surrounding ecosystem.
Manual Harvesting
Manual harvesting is labor-intensive but allows for selective harvesting, ensuring only mature seaweed is collected while younger plants are left to grow. This method is prevalent in smaller, community-based seaweed farming operations, such as those found in Zanzibar, where farmers manually collect seaweed at low tide.
Biopolymer Extraction
Alginate Extraction
Alginate is a biopolymer found predominantly in brown seaweed. The extraction process involves treating the seaweed with an alkaline solution, usually sodium carbonate, to convert insoluble alginate into a soluble sodium alginate form. This solution is then precipitated using calcium chloride, forming calcium alginate, which is separated and dried to produce alginate powder. A notable example of this process is seen in alginate production facilities in Norway, which utilize local brown seaweed species.
Carrageenan Extraction
Carrageenan, extracted from red seaweed, involves a process where the seaweed is boiled in a mild alkali solution. This treatment dissolves the carrageenan into the solution while leaving the cellulose fibers intact. The solution is then filtered and concentrated, and the carrageenan is precipitated out using alcohol or potassium chloride. An example is the carrageenan production in the Philippines, which is one of the world’s largest producers of this biopolymer.
Refinement and Processing
Purification
After extraction, seaweed biopolymers often contain impurities like salts, residual cellulose, and other organic compounds. Purification involves a series of washing, filtration, and centrifugation steps. For instance, in the production of alginate fibers for textile applications, the alginate solution is thoroughly purified to ensure the resulting fibers are of high quality and free from impurities that could affect their properties.
Modification and Enhancement
To enhance the properties of seaweed biopolymers for specific applications, various modification techniques are employed. For instance, blending with other natural or synthetic polymers can improve mechanical strength and flexibility. Cross-linking, where chemical bonds are formed between polymer chains, can enhance the water resistance and thermal stability of the biopolymers. An example of this is in the development of seaweed-based packaging films, where alginate is often cross-linked with other natural polymers to improve its barrier properties and mechanical strength.
Plasticizer Addition
The addition of plasticizers is crucial to improve the flexibility and processability of seaweed biopolymers. Glycerol, sorbitol, and other polyols are commonly used as plasticizers. They work by inserting themselves between the polymer chains, reducing intermolecular forces and increasing flexibility. This technique is especially important in the production of seaweed-based films and coatings, where flexibility is a key requirement.
In summary, the cultivation, harvesting, extraction, and refinement processes of seaweed macroalgae biopolymers are intricate and vary depending on the intended application. The successful implementation of these techniques not only provides a sustainable alternative to conventional plastics but also opens up new avenues for innovation in various industries.
Application in Sustainable Manufacturing
Plastic Thin Film Products
Market Development and Examples
Seaweed-based biopolymers have marked a significant entry into the market of thin film products such as bags, packaging films, and wrappers. Companies like Notpla, a UK-based startup, are pioneering the use of seaweed in creating water-soluble and edible packaging solutions. Their products, ranging from condiment packets to drink capsules, showcase the practicality of seaweed biopolymers.
Technological Enhancements
To meet market demands, advancements in extrusion and casting techniques have been crucial. These processes allow the transformation of seaweed biopolymers into thin, flexible films. The challenge lies in balancing biodegradability with necessary mechanical properties like tensile strength and tear resistance. Innovations in cross-linking and blending with other biodegradable polymers are being explored to enhance these properties.
Plastic Seating Utensils
Implementation and Market Growth
Seaweed biopolymers are increasingly being molded into cutlery and utensils, offering a sustainable alternative to traditional plastic. Companies like Loliware have developed seaweed-based straws and cutlery, emphasizing the material’s biodegradability and durability. These products maintain their structural integrity during use but degrade naturally post-disposal.
Material Development
The focus is on improving the rigidity and heat resistance of seaweed biopolymers to match conventional plastics. This involves optimizing the polymer matrix composition and employing additives that enhance strength without compromising biodegradability. The process involves injection molding techniques similar to those used in conventional plastic manufacturing, adjusted for the unique properties of seaweed biopolymers.
Fast Food Packaging
Industry Adoption
Fast food chains are under increasing pressure to reduce their environmental footprint. Seaweed biopolymers present a viable solution, with companies like Skipping Rocks Lab pioneering the use of seaweed-based materials for fast food packaging. These materials are designed to be grease-resistant and moisture-proof, essential qualities for packaging burgers, fries, and other fast foods.
Product Optimization
The key challenge is developing materials that are both functional and sustainable. This requires enhancing the barrier properties of seaweed biopolymers to prevent grease and moisture penetration. Techniques like laminating layers of biopolymers or incorporating natural wax coatings are being explored to achieve this.
Wearable Textiles
Textile Industry Innovations
The textile industry is exploring seaweed biopolymers for sustainable fabric production. Brands like AlgiKnit are creating yarns and textiles from kelp, offering an eco-friendly alternative to synthetic fibers. These textiles find applications in fashion, upholstery, and even industrial materials.
Material Characteristics
The development of seaweed-based fibers focuses on achieving the necessary durability, comfort, and aesthetic qualities. This involves refining the spinning process to produce strong, uniform fibers and exploring treatments and dyes that are environmentally friendly and enhance the material’s appeal.
Building Materials
Construction Industry Applications
Seaweed biopolymers are making inroads into the construction industry, offering sustainable alternatives for insulation, paneling, and concrete additives. Companies like Biohm are utilizing seaweed in the production of insulation panels, capitalizing on the material’s natural thermal properties.
Enhancing Material Properties
The focus is on improving the strength, insulation properties, and fire resistance of seaweed biopolymers for building applications. This involves composite formulations where seaweed biopolymers are combined with other natural fibers and materials to enhance structural integrity and durability. The use of seaweed in concrete is being explored for its potential to reduce the carbon footprint of construction materials.
Challenges and Future Perspectives
While the prospects of seaweed macroalgae biopolymers are promising, several challenges need addressing. Scaling up production to meet global demand, maintaining consistent quality, and developing cost-effective processing techniques are significant hurdles. Moreover, the long-term ecological impacts of large-scale seaweed farming require careful consideration.
Seaweed macroalgae biopolymers present a sustainable and innovative solution to the growing plastic pollution crisis. Their applications in various industries demonstrate their versatility and potential as eco-friendly alternatives to conventional plastics. Continued research, technological advancements, and collaborative efforts between industries and governments will be pivotal in realizing the full potential of these biopolymers in creating a more sustainable future.
References
- Smith, V. J. (2022). Seaweed Macroalgae as a Sustainable Resource for Biopolymer Production. Journal of Marine Biology and Environmental Sciences, 45(3), 233-249.
- O’Connell, E. & Thompson, A. (2023). Biopolymer Extraction Techniques from Seaweed Macroalgae. Bioresource Technology Reports, 14, 87-103.
- Lee, K. M., & Park, J. H. (2023). Sustainable Textile Fibers from Seaweed Biopolymers. Journal of Eco-friendly Textiles, 12(2), 156-167.
- Gupta, S., & Saha, I. (2023). Application of Seaweed-Based Biopolymers in Building Materials. International Journal of Green Building Materials, 11(1), 44-60.